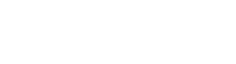
- Login/Register
- Solar System
- Exotic Objects
- Upcoming Events
- Deep-Sky Objects
- Observing Basics
- Telescopes and Equipment
- Astrophotography
- Space Exploration
- Human Spaceflight
- Robotic Spaceflight
- The Magazine

Record-breaking collisions mark start of research at the Large Hadron Collider
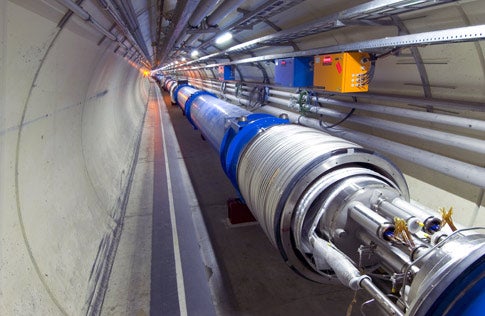
Scientists at the Large Hadron Collider (LHC) at CERN, Geneva, are celebrating after achieving another world first today — proton collisions at 7 trillion electron volts (TeV). This significant milestone, the highest energies ever achieved by a man-made particle accelerator (3.5 TeV per proton beam), marks the start of a 2-year campaign that could see scientists make new discoveries about the universe and answer some of the unresolved questions in physics.
“Achieving collisions at 7 TeV marks the start of a new era in physics research,” said John Womersley, particle physicist and director of science programs at the Science and Technology Facilities Council (STFC), which funds the United Kingdom’s particle physics program. “The LHC aims to explore the nature of the universe just moments after the Big Bang, and it will increase our understanding of how it was created, what it is made of, and how it will evolve. During the coming months, scientists will use data collected at these high energies first to cross-check data and theories from previous experiments, and then to search for particles and forces that we know must exist in the universe but have never been observed. In the next couple of years, this could lead to the discovery of a new law of physics called supersymmetry — which could explain the dark matter that seems to dominate our universe — and even to the discovery of the elusive Higgs boson particle.”
“It’s a great day to be a particle physicist,” said Rolf Heuer, CERN director general. “A lot of people have waited a long time for this moment, but their patience and dedication are starting to pay dividends. The LHC has a real chance over the next 2 years of discovering supersymmetric particles and possibly giving insights into the composition of about a quarter of the universe.”
CERN will run the LHC at 7 TeV for 18 months to 2 years to deliver enough data to the A Toroidal LHC Apparatus (ATLAS), Compact Muon Solenoid (CMS), A Large Ion Collider Experiment (ALICE), and LHC beauty (LHCb)) experiments to make significant advances across a wide range of physics areas. As soon as scientists have rediscovered the known standard model particles, a necessary precursor to looking for new physics, the LHC experiments will then start the systematic search for the Higgs boson.
With the amount of data expected, called one inverse femtobarn by physicists, the combined analysis of ATLAS and CMS will be able to explore a wide mass range, and there’s even a chance of discovering the Higgs boson if it has a mass near 160 GeV (gigaelectron volts). If it’s much lighter or very heavy, it will be more difficult to find in the LHC’s first run.
For supersymmetry, ATLAS and CMS will each have enough data to double today’s existing sensitivity to certain new discoveries. Experiments today are sensitive to some supersymmetric particles with masses up to 400 GeV. Such a high data volume at the LHC pushes the discovery range up to 800 GeV.
Even at the more exotic end of the LHC’s potential discovery spectrum, this LHC run will extend the current reach by a factor of two. LHC experiments will be sensitive to new massive particles, indicating the presence of extra dimensions up to masses of 2 TeV — today’s reach is around 1 TeV.
“There is a whole new landscape of physics to explore at these energies,” said Jon Butterworth from the University College London. “Somewhere in that landscape nature has hidden the way forces are unified and how particles get mass. Today the LHC gets us over the horizon, and we start our exploration!”
“For the last two decades, we have been preparing to make this leap into exploring a new realm of nature,” said Jordan Nash from Imperial College London. “Our studies of the universe and the particles we have been able to produce in accelerators up until now show us that there are important pieces missing in our understanding of the basic building blocks of the universe. Operating the LHC at these energies much higher than ever achieved before will allow us to search for these and find out more about what our universe consists of. Our first taste of data at low- energy operation of the LHC has shown us that our detectors are ready to capture and analyze the data with exquisite precision, and we are all standing by ready to see what nature has in store for us.”
Once the LHC has completed this long run of 18-24 months, there will be a long shutdown of roughly a year during which the LHC will be upgraded and prepared for running at its design energy of 14 TeV. Traditionally, CERN has operated its accelerators on an annual cycle, running for 7 to 8 months with a 4-to-5-month shutdown each year. Being a cryogenic machine operating at very low temperature, the LHC takes about a month to bring up to room temperature and another month to cool down. A 4-month shutdown as part of an annual cycle no longer makes sense for such a machine, so CERN has decided to move to a longer cycle with longer periods of operation accompanied by longer shutdown periods when needed.
“Two years of continuous running is a tall order both for the LHC operators and the experiments, but it will be well worth the effort,” said Rolf Heuer. “By starting with a long run and concentrating preparations for 14 TeV collisions into a single shutdown, we’re increasing the overall running time over the next 3 years, making up for lost time and giving the experiments the chance to make their mark.”
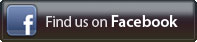
Soar through the Pillars of Creation with NASA’s stunning new video
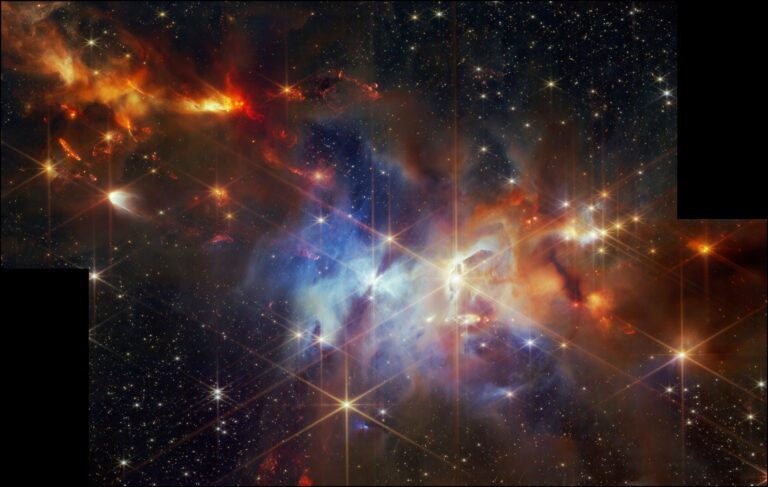
In a first, JWST captures ultra-detailed image of aligned jets in the Serpens Nebula
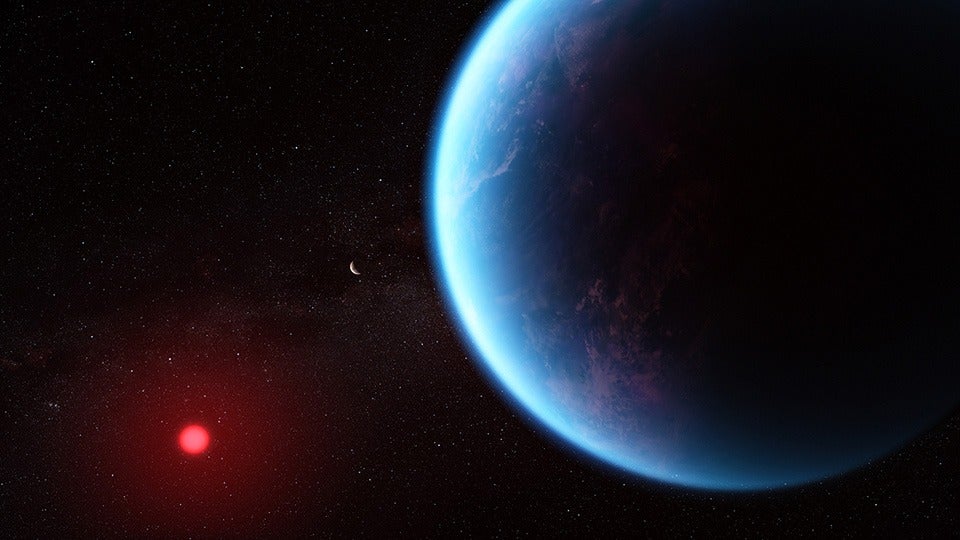
Did we find signs of life on K2-18 b? Not yet, but we might.
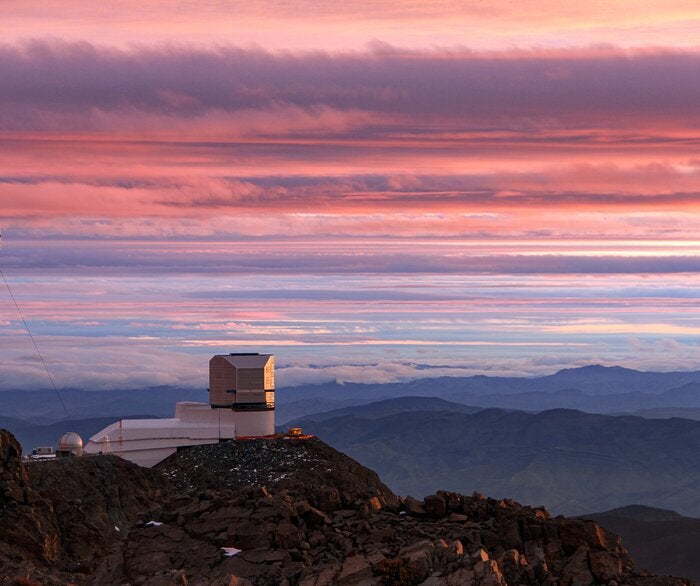
‘It’s going to be awesome’: How the Vera C. Rubin Observatory will survey space and time

2024 Full Moon calendar: When to see the Full Moon and phases
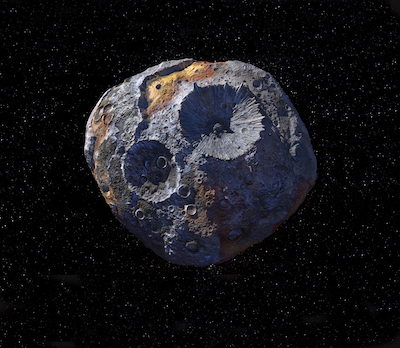
Could Psyche be the core of the planetesimal that struck Earth and formed the Moon?
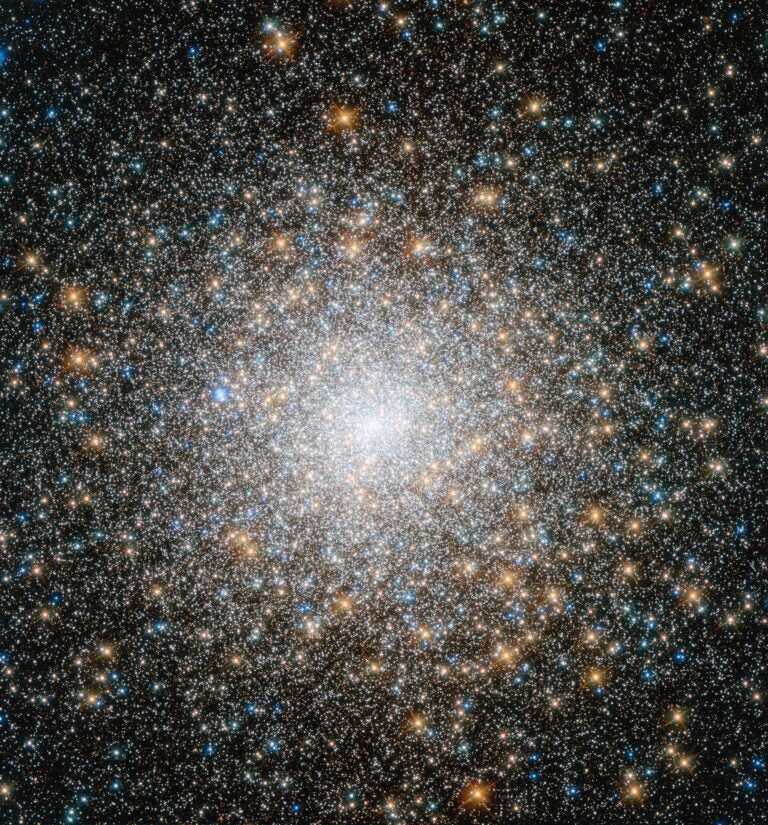
Unusual glimpse of Cosmic Gems could be a breakthrough for astronomers
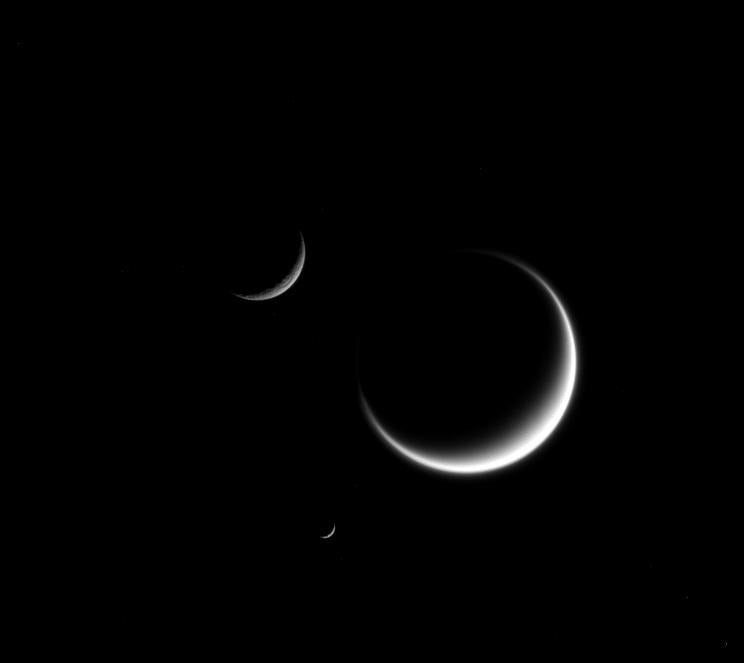
Why does Earth have only one Moon?
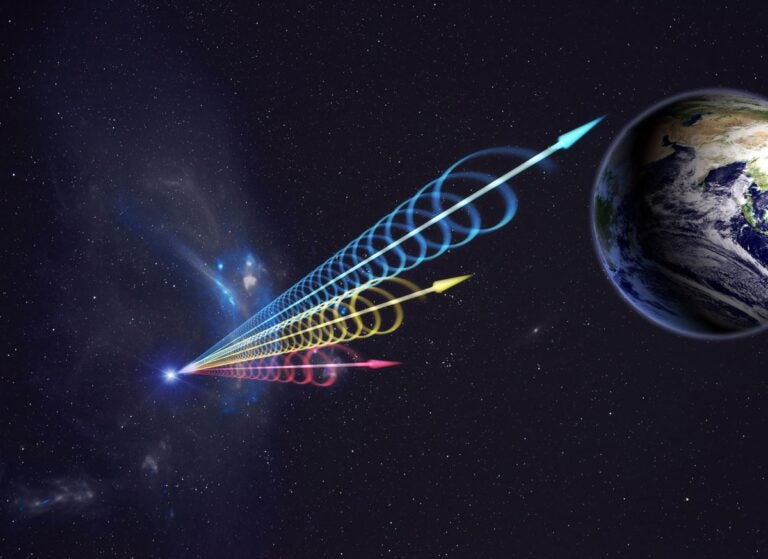
One-shot fast radio bursts may emerge from Milky Way-like galaxies, new research suggests
- New Hampshire
- North Carolina
- Pennsylvania
- West Virginia
- Online hoaxes
- Coronavirus
- Health Care
- Immigration
- Environment
- Foreign Policy
- Kamala Harris
- Donald Trump
- Mitch McConnell
- Hakeem Jeffries
- Ron DeSantis
- Tucker Carlson
- Sean Hannity
- Rachel Maddow
- PolitiFact Videos
- 2024 Elections
- Mostly True
- Mostly False
- Pants on Fire
- Biden Promise Tracker
- Trump-O-Meter
- Latest Promises
- Our Process
- Who pays for PolitiFact?
- Advertise with Us
- Suggest a Fact-check
- Corrections and Updates
- Newsletters
Stand up for the facts!
Our only agenda is to publish the truth so you can be an informed participant in democracy. We need your help.
I would like to contribute
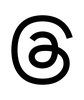
- Facebook Fact-checks
- Threads posts
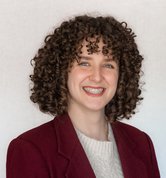
We feel fine: CERN scientists did not end the world 12 years ago. This claim is satire.
If your time is short.
The story originated on a satire site.
Experiments in 2012 with a particle accelerator led to the discovery of the Higgs boson particle, and earned CERN researchers a Nobel Prize.
Was R.E.M. onto something in 1987 when they sang, "It's the end of the world as we know it?" On June 18, a Threads post shared a screengrab of what appeared to be a miscapitalized news headline that read, "‘Sorry, but we accidentally ended the world in 2012’ Admits CERN scientists."
The screengrab also included a picture of CERN scientists on a panel and the text of a story that says scientists had admitted to "ending the world as we know it back in 2012, while performing experiments into the Higgs boson particle."
The Threads post was flagged as part of Meta’s efforts to combat false news and misinformation on its News Feed. (Read more about our partnership with Meta , which owns Threads, Facebook and Instagram.)
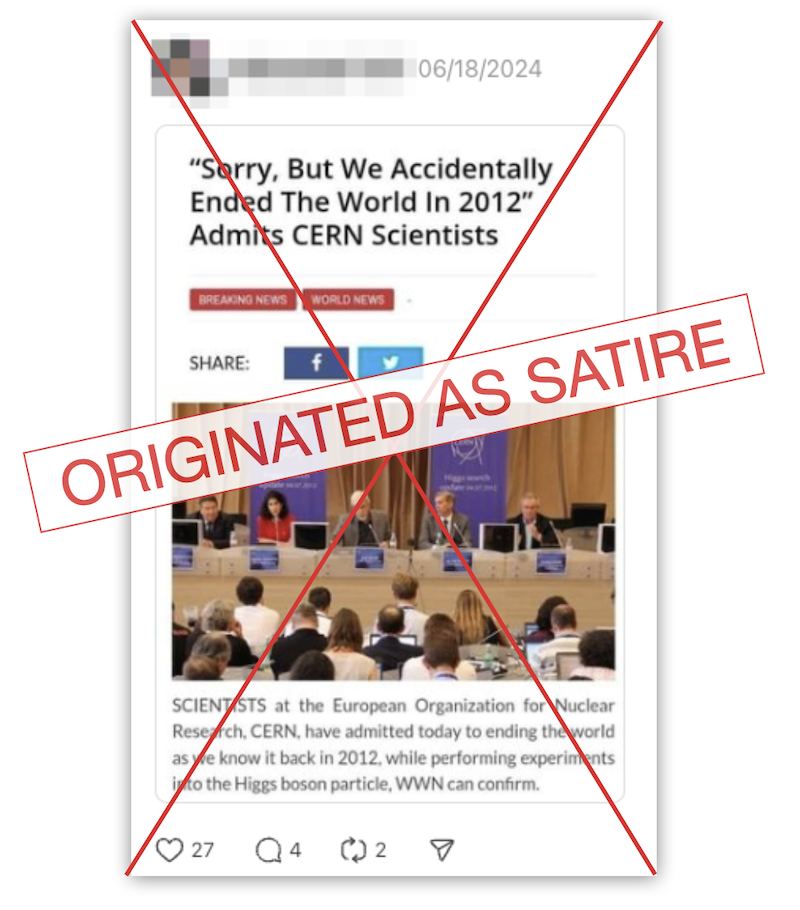
The image in the post is a screenshot of a story published on Waterford Whispers News, a self-described "satirical newspaper." We have previously fact-checked other claims that have originated on this website but been reshared without that context.
Featured Fact-check
The satirical story’s text claims that "several key scientists" at a Switzerland research facility apologized for a "terrible accident that has only come to light now." The accident? That the discovery of the Higgs boson particle "inadvertently shifted the entire planet into an alternate reality," and that "none of us technically exist."
The European Organization for Nuclear Research, aka CERN — derived from the French name "Conseil Européen pour la Recherche Nucléaire" — primarily researches particle physics, which the group’s website describes as "the study of the fundamental constituents of matter and the forces acting between them." CERN is home to the Large Hadron Collider, the most powerful particle accelerator in the world . In the Large Hadron Collider, two beams of particles travel in opposite directions at nearly light speed and are made to collide.
In 2012, Large Hadron Collider experiments led the discovery of the Higgs boson particle , named for British physicist Peter Higgs. In the 1960s, Higgs postulated about the existence of a particle that interacted with other particles at the beginning of time to provide them with their mass. In 2013 CERN researchers won a Nobel Prize in physics for their discovery. But that particle did not shift us into a new reality.
We have previously fact-checked whether CERN’s activation of its Large Hadron Collider was connected to the April 8 total solar eclipse and rated it False . CERN’s website’s’ frequently asked questions addresses such queries as, "Will CERN open a door to another dimension?" and "Will CERN generate a black hole?"
We rate the claim that CERN scientists admit they "accidentally" ended the world in 2012 False.
Read About Our Process
The Principles of the Truth-O-Meter
Our Sources
Threads post , June 18, 2024
Waterford Whispers News, "Sorry, But We Accidentally Ended The World In 2012" Admits CERN Scientists ," ( archived ), accessed June 26, 2024
Waterford Whispers News, " Disclaimer ," ( archived ), accessed June 26, 2024
PolitiFact, " No, NASA has not communicated with aliens ," June 25, 2018
PolitiFact, " No, North Korea didn’t ‘land’ someone on the sun ," Sept. 12, 2022
CERN, " What is the universe made of? How did it start? Physicists at CERN are seeking answers, using some of the world's most powerful particle accelerators ," accessed June 26, 2024
CERN, " CERN FAQ: LHC: The guide ," accessed June 26, 2024
CERN, " FAQs ," accessed June 26, 2024
Britannica, " Large Hadron Collider (LHC) | Definition, Discoveries, & Facts ," May 31, 2024
Nobel Prize, " The Nobel Prize in Physics 2013 ," accessed June 26, 2024
PolitiFact, " Is CERN activating the world’s most powerful particle accelerator for the April 8 eclipse? No ," April 5, 2024
Browse the Truth-O-Meter
More by grace abels.

Support independent fact-checking. Become a member!

- June 25, 2024 | Black Holes and Dark Revelations: Gravitational Waves Provide New Clues to the Composition of Dark Matter
- June 25, 2024 | Quantum Surprises in Ultra-Clean Materials Redefine Metal Physics
- June 25, 2024 | Meet the Virus-Killing Plastic That’s Changing the COVID Game
- June 25, 2024 | Quantum Transformation: TiS3 Nanoribbons Become Superconductors Under Pressure
- June 25, 2024 | How CBD and Metformin Are Revolutionizing Treatment for Autism-Related Behavioral Issues
Einstein’s “Spooky Action at a Distance” Between the Heaviest Particles at the Large Hadron Collider
By CMS Collaboration June 17, 2024
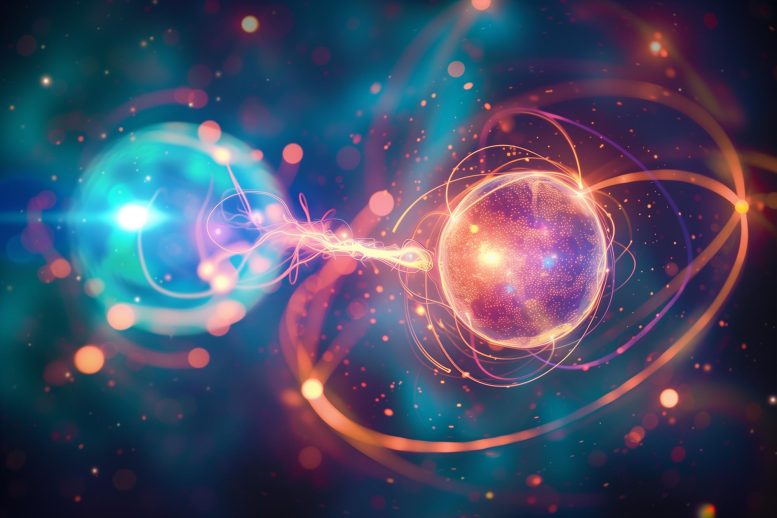
The ATLAS and CMS experiments have observed entanglement between top quarks and their antiparticles, confirming this phenomenon at high energies. This opens new avenues for quantum research, supported by large data samples from the LHC and enhanced by modern analytical techniques. Credit: SciTechDaily.com
Two decades after the first proof of photon entanglement, the ATLAS and CMS experiments have observed quantum entanglement between top quarks and their antiparticles at the Large Hadron Collider.
This breakthrough confirms entanglement at high energies and offers a new perspective on quantum mechanics. Meanwhile, the vast number of top quark pairs produced at the LHC allows for extensive studies, further bolstered by advanced machine learning techniques in measuring spin entanglement and systematic uncertainties.
Quantum Entanglement in Particle Physics
Recently, and about two decades after the first solid proof of entanglement between two photons by Anton Zeilinger and his team, the ATLAS and CMS experiments reported the observation of quantum entanglement between the top quark and its antiparticle simultaneously produced at rest at the Large Hadron Collider.
Confirming the quantum entanglement between the heaviest fundamental particles, the top quarks, has opened up a new avenue to explore the quantum nature of our world at energies far beyond what is accessible, e.g., in quantum optics. At the same time, the large production rate of top quark pairs at the Large Hadron Collider provides a gigantic data sample of top quarks, offering a unique opportunity for these studies.
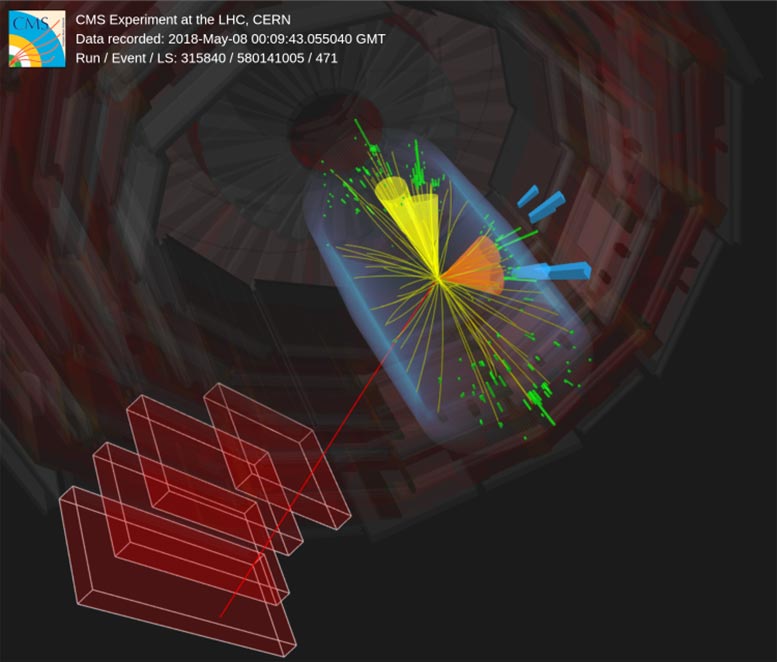
Quantum entanglement between top quarks and antiparticles has been confirmed at the LHC, marking a significant advancement in high-energy quantum physics, supported by extensive data and advanced analysis methods. Credit: CERN
Einstein’s Challenge to Quantum Mechanics
In quantum mechanics, two particles are entangled if we know the state of one of them when measuring the other. This is true even if the two initially entangled particles are placed very far away from each other before the measurement. This is what Einstein referred to as “spooky action at a distance”: although the information cannot travel faster than the speed of light, the second particle is guaranteed to be found in a corresponding state instantly when the measurement of the first one is performed. In 1934, Einstein and his collaborators proposed a thought experiment that, according to them, was exposing the inconsistency of quantum mechanics.
To solve the paradox, they suggested that our description of entanglement is incomplete and that there are other quantities at play in the system that we cannot access experimentally. Entanglement would then be the result of our ignorance about these hidden variables.
Advanced Techniques in Measuring Entanglement
In a new measurement, the CMS collaboration examines, for the first time, the spin entanglement of a top quark and a top antiquark that are simultaneously produced at a very high speed with respect to each other. The two particles are therefore far apart before decaying, i.e., their distance is larger than what can be covered by information transferred at the speed of light. The correlation between the spins of the quark and of the antiquark is measured by looking at the angular distributions of their decay products.
The analysis brings into play state-of-the-art machine learning methods to correctly assign the top (anti)quark decay products and to improve the modeling of systematic uncertainties. The observed levels of entanglement, characterized by the parameter Δ E , are shown in Fig. 1 for two different kinematic regions.
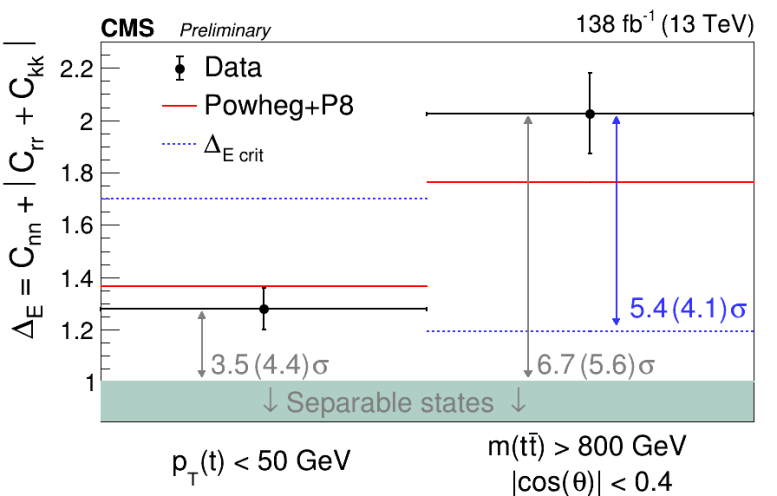
Figure 1: The observed levels of entanglement characterized by ΔE are shown in two kinematic regions. The measurements (points) are shown with their uncertainties and compared to the SM predictions (red lines). The horizontal blue lines correspond to the maximum level of entanglement ΔE critical that can be explained by the exchange of information between the quark and the antiquark at the speed of light. The first bin corresponds to top quarks produced with transverse momentum less than 50 GeV, while in the last bin the top quark pairs have high invariant mass, i.e., are moving with a large velocity with respect to each other. The measured ΔE is greater than 1 in both kinematic regions, confirming entanglement between the two particles. In the second bin, in particular, the top quark-antiquark pairs are moving at such a large relative speed that only in 10% of the cases they would have a chance to communicate. There, the entanglement is significantly higher than ΔE critical, which is the level of entanglement that could be explained by information exchange via hidden variables at the speed of light. Therefore, the measurement shows that there is indeed “spooky action at a distance” between the heaviest known particles. Credit: CERN

More on SciTechDaily
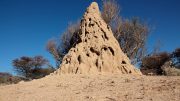
“Astonishing” Discovery – 34,000-Year-Old Inhabited Termite Mounds Discovered in South Africa
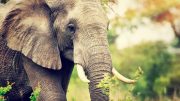
Ivory Coast Without Ivory? Massive Extinction of African Forest Elephants in Côte d’Ivoire
Scientists have created a comfortable vest that will fix your posture.
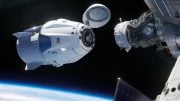
Watch It Live: NASA Coverage for Private Astronaut Axiom Mission 1 Departure From Space Station
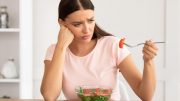
Vegetarians More Likely To Be Depressed Than Meat-Eaters – Here’s the Science Behind It
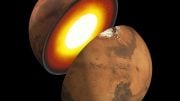
NASA InSight Lander Takes Deep Mars Measurements: Seismograph Data Reveals Boundaries From Crust to Core
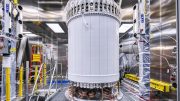
Dark Matter Search Project – Using a Time Projection Chamber Nearly a Mile Underground – Carefully Ramps Up Science Work
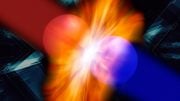
Largest Matter-Antimatter Asymmetry Observed at Large Hadron Collider
1 comment on "einstein’s “spooky action at a distance” between the heaviest particles at the large hadron collider".
Outdated theories may mislead researchers. Artificial intelligence based on topological vortex self-organization (including chip manufacturing, battery construction, and communication methods) is the direction of human scientific development. Despite the hardships of scientific research, there have always been different landscapes along the way. The physical phenomena observed by researchers are always superficial, not the natural essence of things. The natural essence of things needs to be refined and sublimated via the phenomena observed in experiments. Learning widely from others’ strong points may inspire you. If researchers are willing to further consider, you can browse https://zhuanlan.zhihu.com/p/701333717 and https://zhuanlan.zhihu.com/p/463666584 . Good luck to the researchers.
Leave a comment Cancel reply
Email address is optional. If provided, your email will not be published or shared.
Save my name, email, and website in this browser for the next time I comment.
CERN Accelerating science
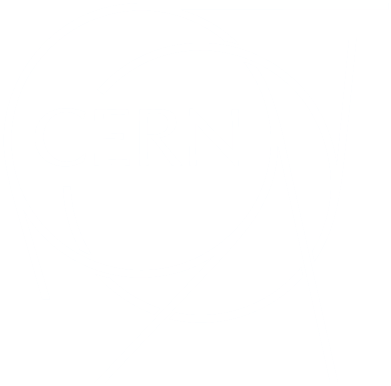
Future colliders and fusion reactors
CERN and EUROfusion are collaborating to develop innovative technologies for future colliders and nuclear fusion reactors
11 June, 2024
By Antoine Le Gall Anaïs Schaeffer
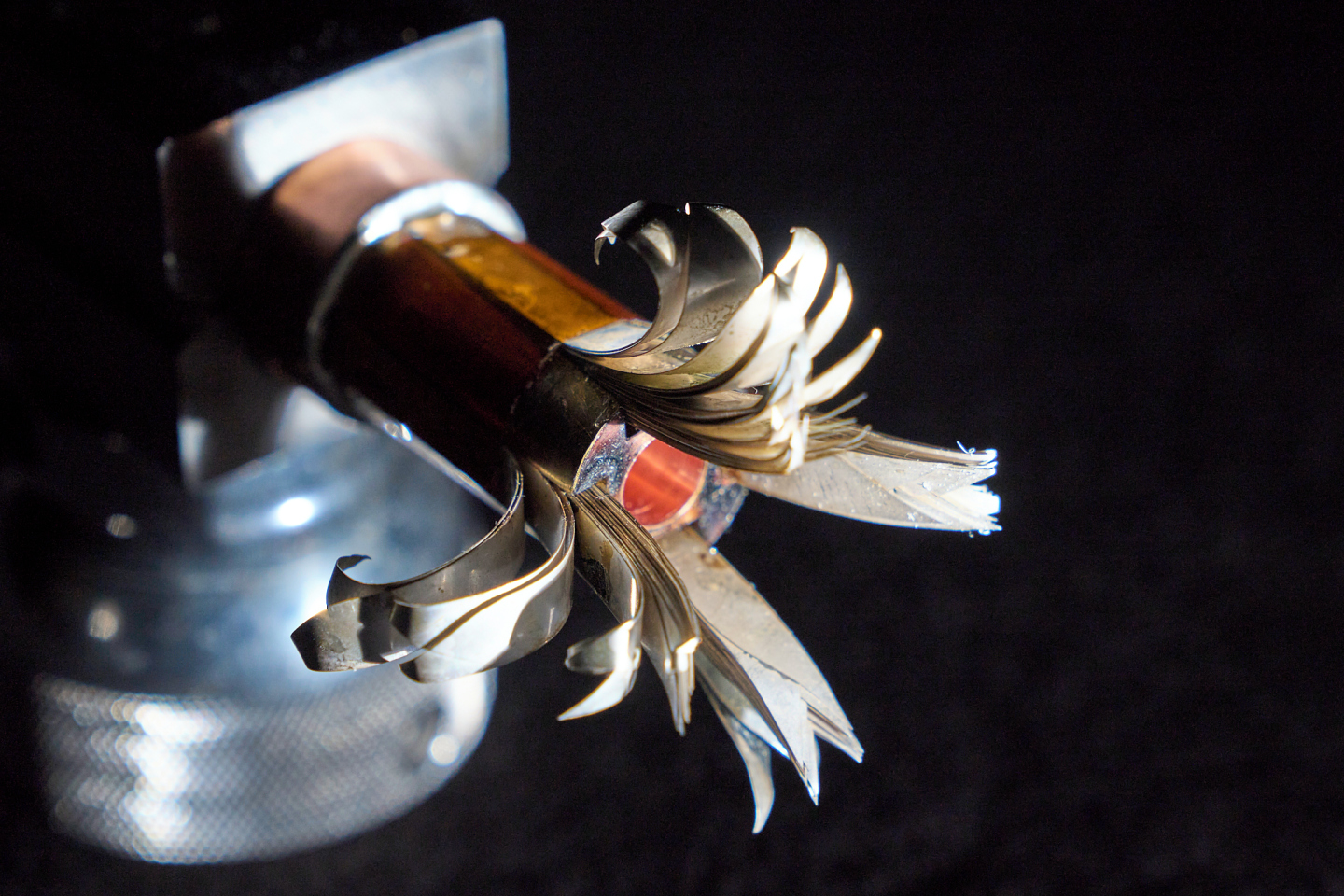
This high-temperature superconducting (HTS) cable, developed at CERN, is inspired by those developed for advanced nuclear fusion reactor concepts. It consists of a hollow copper profile with twisted grooves containing stacks of soldered HTS tapes (shown opened in a "flower" shape in the image). (Image: CERN)
CERN's accelerator experts and EUROfusion's nuclear fusion specialists are now working jointly to develop innovative technologies for future colliders and nuclear fusion reactors, drawing on their respective unique competencies, in particular in the area of high field magnets.
The common projects are facilitated by the collaboration agreement that was signed in November 2023 by CERN and members of EUROfusion , the European consortium of fusion research laboratories carrying out a technical design of a fusion demonstration power plant (DEMO) to succeed ITER .
Marking a milestone in scientific cooperation, this partnership paves the way for joint ventures in a broad spectrum of areas, encompassing research and development in physics, engineering and technology. It focuses on the engineering design and construction of significant scientific experiments and instruments.
CERN established a dedicated fusion technology coordination unit in 2023 with the involvement of accelerator and knowledge transfer experts, reflecting the Organization’s commitment to fostering collaboration across multiple scientific disciplines. The agreement signed between EUROfusion and CERN is a prominent example of the unit’s activities.
“There are clear synergies in the development of technologies for nuclear fusion and those for future colliders, particularly with regard to the use of high-temperature superconducting (HTS) conductors. In the ideation of DEMO, the demonstration fusion power plant that will succeed ITER, the choice of tokamak central solenoids using HTS materials is being explored by a number of EUROfusion members”, says Gianfranco Federici, Head of the EUROfusion Fusion Technology Department. “The collaboration agreement provides a platform for all of our members and CERN to collaborate and create a centre of excellence for fusion technology”.
Central to this alliance, the inaugural project initiated this year is envisioned as a crucial step towards a future testbed for tritium blanket technology. It is scheduled for completion by the end of 2024. “The teams at CERN and EUROfusion are engaging in fruitful exchanges concerning magnet concepts and designs based on high-temperature superconductors. The extraordinary challenges posed by the magnets of a muon collider require the development of new concepts, some of them similar to those of fusion machines. This is particularly true for the target solenoid, a key component of the collider, which needs to reach a very high field, is subjected to large heat and radiation loads and is likely to be built with HTS conductors”, says Luca Bottura, who is leading the magnet design efforts of the International Muon Collider Collaboration and the magnet work package of the EU MuCol design study .
Recently, the scope of the collaboration has been extended to include cooperation on advanced shielding materials, of interest both to fusion and accelerators. This topic will be the focus of a joint workshop, which is due to take place later this year.
Related Articles
How well do you know the cern & society found..., hilumi news: the hl-lhc’s cold powering syste..., cern 70th anniversary exhibition at geneva ai..., also on knowledge sharing, cern's communications receive european recogn..., instruments of vision opens in santiago de co..., cern welcomes international year of quantum s..., unveiling the science of tomorrow: fcc study ..., the importance of dialogue for future project..., adding cern to the shopping list, cern and enea plan to develop liquid-metal te....
Physical Review Accelerators and Beams
- Special Editions
- Editorial Team
- Open Access
Energy deposition studies for the Upgrade II of LHCb at the CERN Large Hadron Collider
Alessia ciccotelli, robert b. appleby, francesco cerutti, kevin buffet, francois butin, gloria corti, luigi salvatore esposito, ruben garcia alia, matthias karacson, giuseppe lerner, maud wehrle, and daniel prelipcean, phys. rev. accel. beams 27 , 061003 – published 24 june 2024.
- No Citing Articles
- INTRODUCTION
- PROVISIONAL SCHEDULE FOR POST-UPGRADE II…
- SIMULATION MODELING OF IR8 AND LHCb…
- IMPLICATIONS ON THE LHCb DETECTOR
- IMPLICATIONS ON THE LHC ELECTRONIC…
- IMPLICATIONS ON THE LHC MAGNETS
- CONCLUSIONS AND OUTLOOK
- ACKNOWLEDGMENTS
The Upgrade II of the Large Hadron Collider beauty (LHCb) experiment is proposed to be installed during the CERN Long Shutdown 4, aiming to operate LHCb at 1.5 × 10 34 cm − 2 s − 1 that is 75 times its design luminosity [1] and reaching an integrated luminosity of about 400 fb − 1 by the end of the High Luminosity LHC era. This increase of the data sample at LHCb is an unprecedented opportunity for heavy flavor physics measurements. A first upgrade of LHCb (Upgrade I), completed in 2022, has already implemented important changes of the LHCb detector and, for the Upgrade II, further detector improvements of the tracking system, the particle identification system and the online and trigger infrastructure are being considered. Such a luminosity increase will have an impact not only on the LHCb detector but also on the LHC magnets, cryogenics, and electronic equipment placed in the insertion region 8. In fact, the LHCb experiment was conceived to work at a much lower luminosity than ATLAS and CMS, implying minor requirements for protection of the LHC elements from the collision debris, and therefore, a different layout around the interaction point. The Upgrade I has already implied the installation of an absorber for the neutral particle debris (TANB). However, the luminosity target proposed for this second upgrade requires to review the layout of the entire insertion region in order to ensure safe operation of the LHC magnets and to mitigate the risk of failure of the electronic devices. The objective of this paper is to provide an overview of the implications of the Upgrade II of LHCb in the experimental cavern and in the tunnel with a focus on the LHCb detector, electronic devices, and accelerator magnets. This proves that the Upgrade II luminosity goal can be sustained with the implementation of protection systems for magnets and electronics. The electronics placed in the experimental areas and in the cavern needs to be protected to mitigate a single event effect risk, which may imply recurring downtime of the LHC. On the other hand, protection for the first quadrupole of the final focus triplet and for the separation dipole are needed to prevent their quench and to reach the desired lifetime. Moreover, the normal-conducting compensators require the installation of shielding to limit their head coil degradation.
- Received 26 October 2023
- Accepted 1 May 2024
DOI: https://doi.org/10.1103/PhysRevAccelBeams.27.061003

Published by the American Physical Society under the terms of the Creative Commons Attribution 4.0 International license. Further distribution of this work must maintain attribution to the author(s) and the published article’s title, journal citation, and DOI.
Published by the American Physical Society
Physics Subject Headings (PhySH)
- Physical Systems
Authors & Affiliations
- The University of Manchester and the Cockcroft Institute, Department of Physics and Astronomy, School of Natural Sciences, Oxford Road, Manchester M13 9PL, United Kingdom and CERN , CH 1211 Geneva 23, Switzerland
- The University of Manchester and the Cockcroft Institute, Department of Physics and Astronomy, School of Natural Sciences, Oxford Road, Manchester M13 9PL, United Kingdom
- CERN , CH 1211 Geneva 23, Switzerland
- CERN , CH 1211 Geneva 23, Switzerland and Technical University of Munich (TUM) , Arcisstraße 21, 80333 München, Germany
- * [email protected]
Article Text
Vol. 27, Iss. 6 — June 2024
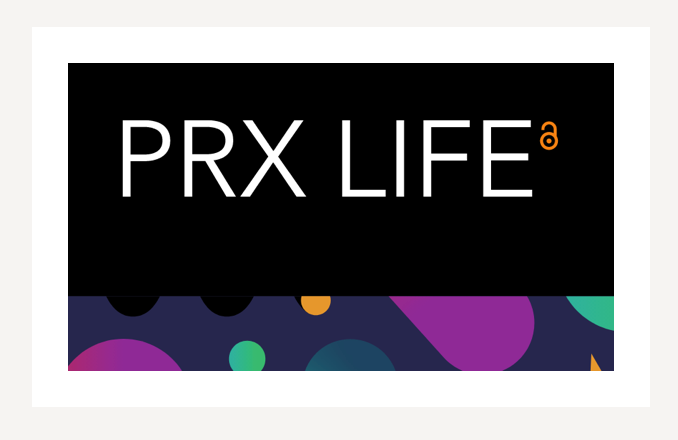
Authorization Required
Other options.
- Buy Article »
- Find an Institution with the Article »
Download & Share
Luminosity performances in IR8 with and without the Upgrade II of LHCb [ 2, 3, 7 ].
Top view at beam height of the fluka geometry of the LHCb cavern and surrounding areas.
Schematic side-view of the Upgrade II detector extracted from the Technical Design Report (TDR) published by the LHCb Collaboration [ 3 ].
Top view of annual HEH fluence (averaged in the third dimension between − 1.1 and 1.1 m with respect to the beam height) during Run 5, assuming the same IR8 layout as in Runs 3–4 and an annual luminosity of 50 fb − 1 . The simulation assumed upward polarity of the LHCb spectrometer and an external horizontal crossing of 250 μ rad , pointing inside the ring, at s = 14 TeV .
Top view of the R -factor map at beam height.
3D fluka geometry of UX85 and US85, including the LHCb detector and the shielding wall.
3D fluka geometry of the shielding wall seen from the LHCb experiment side.
Top view of annual HEH fluence (averaged in the third dimension between − 1.1 and 1.1 m with respect to the beam height) during Run 5 in the presence of the shielding wall. The same simulation conditions as in Fig. 4 apply.
Cross-sectional view of annual HEH fluence (averaged along the beam direction between 13 and 17 m from IP8) after the Upgrade II of LHCb with the current layout (left plot) and with the implementation of the shielding wall (right plot). The same simulation conditions as in Fig. 4 apply.
Transverse dose distribution at the maximum of the IP-face front coils of the indicated conventional compensator magnets. External upward vertical crossing is assumed and values are normalized to 360 fb − 1 . The bottom plot shows the geometry apertures around IP8, highlighting the location of the compensator magnets and the LHCb spectrometer in red and the shielding walls in gray.
Three-dimensional dose maps plotted on a longitudinal cutaway of the right short compensator magnet for the current geometry (left) and with the addition of a tungsten shielding with a thickness of 4 cm (right). Values are normalized to 360 fb − 1 . Upward external vertical crossing is assumed. The scoring mesh is Cartesian with transverse and longitudinal resolution of 3 mm.
Transverse peak dose profiles on the front upper coil of the MBXWS on the left side of IP8, evaluated with a longitudinal resolution of 5 cm for different shielding options, implemented (if any) after accumulating 60 fb − 1 during Run 3 and Run 4. Upward external crossing and downward polarity of the LHCb spectrometer have been assumed.
Layout of the triplet on the right side of IP8.
Longitudinal profile of peak power density in the superconducting coils along the triplet and the D1 on the right side of IP8 (IP1 for the red curve), which is at z = 0 . Values are averaged over the cable radial thickness and the azimuthal resolution is of 2°. Two curves, normalized to 1.5 × 10 34 cm − 2 s − 1 , refer to the LHCb Upgrade II operational scenario with s = 14 TeV , external vertical crossing, and either upward (blue crosses) and downward (green squares) polarity of the LHCb spectrometer. The red curve refers to the ATLAS insertion (where no superconducting D1 is present) and an operational Run 3 scenario with s = 13.6 TeV at 2 × 10 34 cm − 2 s − 1 . The red dashed line represents the quench limit for the triplet quadrupoles and the black dashed line represents the design limit [ 27 ].
Longitudinal profile of peak dose in the superconducting coils along the triplet and the D1 on the right side with respect to IP8 (at z = 0 ). The azimuthal and radial resolution is of 2° and 3 mm, respectively. External vertical crossing has been considered for s = 14 TeV . An integrated luminosity of 360 fb − 1 is assumed to be collected half with either polarity of the LHCb spectrometer. The gray curve is for the current layout, while the black one combines 300 fb − 1 with the shielding options discussed in Secs. 6b1 and 6b2 and 60 fb − 1 without any mitigation measure up to the end of Run 4. The red dashed line represents the design lifetime of Q1–Q3 [ 28 ] and D1, while the green one represents the design lifetime of the correctors [ 29 ].
Front (left) and inside (right) view of MBXWS-TAS. The pink blocks are made of tungsten. The shielding to protect its own front coils on the vertical plane is included.
Peak power density along the superconducting coils of the Q1 on the right side with respect to IP8 (at z = 0 ). External vertical crossing and downward polarity of the LHCb spectrometer have been assumed. The black points correspond to the ones reported in green in Fig. 14 . The red squares represent the scenario featuring an ideal MBXWS-TAS equipped of tungsten in contact with the beam pipe, over a transverse width of 10 cm. The blue triangles correspond to a more realistic MBXWS-TAS design in which a 3 mm gap is left between the pipe and the absorber, to accommodate the bakeout tape, and the absorber transverse width is increased to 24 cm. The green crosses refer to a 10 mm reduction of the vacuum aperture diameter, enabling the shielding extension at smaller radii. Resolution and normalization are as in Fig. 14 .
Peak power density along the superconducting coils of the D1 on the right side with respect to IP8 (at z = 0 ). External vertical crossing and downward polarity of the LHCb spectrometer have been assumed. The black points correspond to the ones reported in green in Fig. 14 . The red squares represent the scenario featuring the proposed replacement of the D1 beam screen allowing for the increase of the stainless steel cold bore wall thickness by 5.5 mm. The blue triangles are obtained extending upstream the thick cold bore up to the Q3 non-IP end. Resolution and normalization are as in Fig. 14 .
3D view (from the inside of the ring) of the fluka geometry on the left side of IP8 including the recombination dipole D2, the TANB absorber (installed during LS2), and the horizontal and vertical tertiary collimators, which are installed on the incoming (external) beam vacuum chamber.
Longitudinal profile of peak power density in the D2 superconducting coils on the left side of IP8 (at z = 0 ). Values are averaged over the cable radial thickness and normalized to 1.5 × 10 34 cm − 2 s − 1 . The azimuthal resolution is of 2°. External vertical crossing has been considered for s = 14 TeV with either upward (red points) and downward (black points) polarity of the LHCb spectrometer.
Power density distribution on the IP-face of D2 for upward (top) and downward (bottom) polarity of the LHCb spectrometer in the case of external vertical crossing.
Transverse dose distribution at the longitudinal maximum in the D2 superconducting coils of the outgoing beam on the left side of IP8 for external vertical crossing. An integrated luminosity of 360 fb − 1 is assumed to be collected half with either LHCb polarity.
Longitudinal profile of peak dose in the superconducting coils of the magnets of the left DS, assuming an integrated luminosity of 360 fb − 1 collected half with either polarity of the LHCb spectrometer, for external vertical crossing.
Sign up to receive regular email alerts from Physical Review Accelerators and Beams
Reuse & Permissions
It is not necessary to obtain permission to reuse this article or its components as it is available under the terms of the Creative Commons Attribution 4.0 International license. This license permits unrestricted use, distribution, and reproduction in any medium, provided attribution to the author(s) and the published article's title, journal citation, and DOI are maintained. Please note that some figures may have been included with permission from other third parties. It is your responsibility to obtain the proper permission from the rights holder directly for these figures.
- Forgot your username/password?
- Create an account
Article Lookup
Paste a citation or doi, enter a citation.
CERN Accelerating science
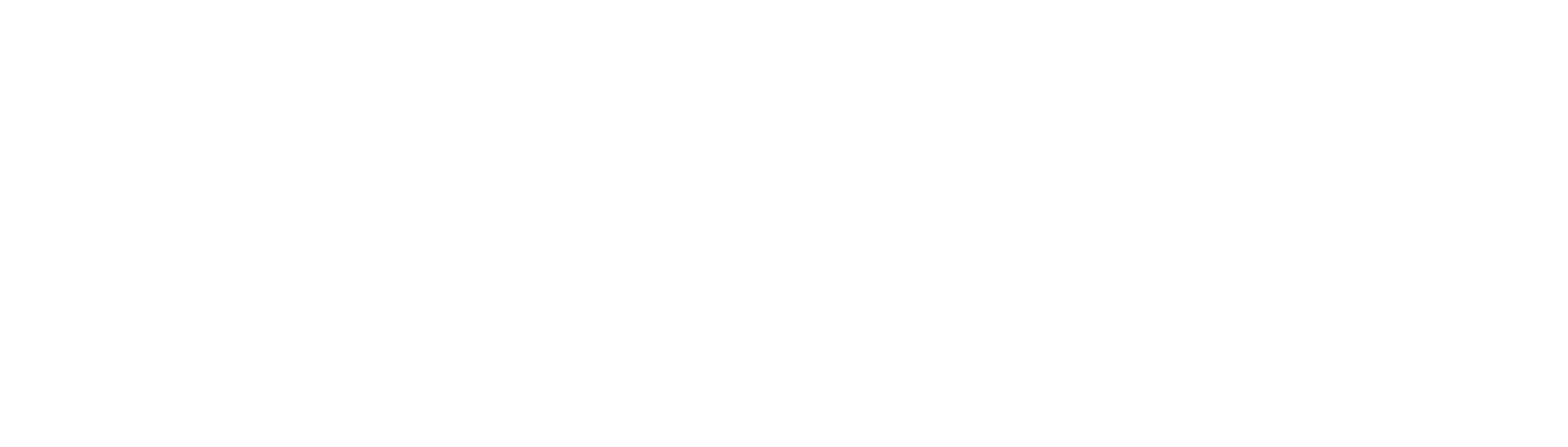
Large Hadron Collider Tech Might Help Self-Driving Cars "See" the Road | MSN Auto
Athree-year study between Volvo’s autonomous software arm and the Large Hadron Collider in Europe shows promise in helping self-driving cars understand the road and make split decisions.
The Large Hadron Collider is undeniably epic. It’s the world’s largest and most powerful particle accelerator capable of smashing particles together at nearly the speed of light using superconducting magnets. After the collision, physicists take a look at the extremely short-lived wreckage to discover the building blocks of our universe. The LHC is run by CERN, or the European Council for Nuclear Research (Conseil Européen pour la Recherche Nucléaire) and has been accelerating particles since 2008.

IMAGES
VIDEO
COMMENTS
March 30, 2010. Scientists at the Large Hadron Collider (LHC) at CERN, Geneva, are celebrating after achieving another world first today — proton collisions at 7 trillion electron volts (TeV).
CERN is home to the Large Hadron Collider, the most powerful particle accelerator in the world. In the Large Hadron Collider, two beams of particles travel in opposite directions at nearly light ...
CERN conducts one of the world’s largest science experiments and the facility houses the world’s largest particle accelerator, the Large Hadron Collider (LHC). We had a discussion and tour of ...
The Large Hadron Collider ( LHC) is the world's largest and highest-energy particle collider. [1] [2] It was built by the European Organization for Nuclear Research (CERN) between 1998 and 2008 in collaboration with over 10,000 scientists and hundreds of universities and laboratories across more than 100 countries. [3]
Einstein’s “Spooky Action at a Distance” Between the Heaviest Particles at the Large Hadron Collider. The ATLAS and CMS experiments have observed entanglement between top quarks and their antiparticles, confirming this phenomenon at high energies. This opens new avenues for quantum research, supported by large data samples from the LHC ...
CERN, located near Geneva, Switzerland, is the world’s largest particle physics laboratory. Production of top quarks requires very high energies accessible at the Large Hadron Collider ...
CERN's accelerator experts and EUROfusion's nuclear fusion specialists are now working jointly to develop innovative technologies for future colliders and nuclear fusion reactors, drawing on their respective unique competencies, in particular in the area of high field magnets. CERN Director for Accelerators and Technology, Mike Lamont, and EUROfusion Chair (presently Programme Manager ...
Description. We travel to the heart of the most famous and probably least understood scientific research center in the world—CERN. We delve into the very heart of the Large Hadron Collider to meet the scientists behind one of the biggest discoveries of the past 10 years, the Higgs Boson, we explore how the science at CERN is spinning out into the wider world and get a sneak peek at their new ...
The Upgrade II of the Large Hadron Collider beauty (LHCb) experiment is proposed to be installed during the CERN Long Shutdown 4, aiming to operate LHCb at 1.5 × 10 34 cm − 2 s − 1 that is 75 times its design luminosity [1] and reaching an integrated luminosity of about 400 fb − 1 by the end of the High Luminosity LHC era.
Athree-year study between Volvo’s autonomous software arm and the Large Hadron Collider in Europe shows promise in helping self-driving cars understand the road and make split decisions. The Large Hadron Collider is undeniably epic.